Scroll to the bottom to watch the YouTube tutorial.
Why are there 3 blades? Why are they so high? Why are they so slow? and How does it even generate electricity? Grab a pen and paper to make notes, sip from your engineering mindset mug. Lets find out. You can get your mug HERE.
This basic wind turbine can power a small LED. This larger one can power a small home, but these mega turbines can power entire towns.
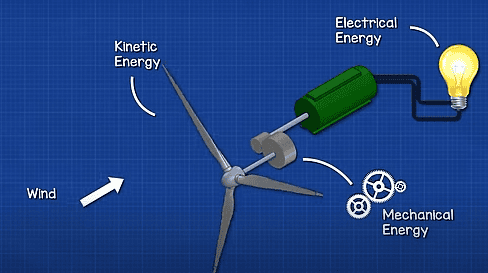
A wind turbine simply converts the kinetic energy of the wind into mechanical energy, and that is converted into electrical energy.
We can feel the energy of the wind on our hand. We know it can turn a windmill. We can turn that into motion, like this strange wind powered walking …. thing. Or, we can attach a generator to it, and it will produce electricity.
Try it yourself, take a simple DC motor. Spin the shaft and you will notice it produces a voltage. So just attach a blade to it, and it’ll spin in the wind and generate electricity.
The speed of the wind increases the higher we go and it’s also less turbulent. The larger the blades, the more wind energy we can capture. Large blades need to be higher off the ground. But the speed of the wind is the largest influencer in power generation.
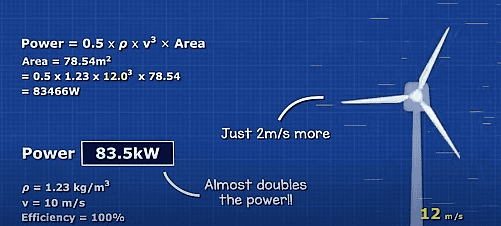
Large turbines are difficult to transport, so we often find the largest turbines out at sea where space isn’t a problem. Although it’s a lot cheaper and easier to install them on land. But they do mark the landscape, they cast long flickering shadows and can also create some noise.
The wind turbines need a deep, strong foundation. We can extend down into the sea bed, but some waters are so deep, it’s easier to just float the wind turbine on a platform.
You might notice that smaller wind turbines have a tail fin at the back, but large ones don’t. I’ll explain why later in the article.
The wind turbine needs to face the wind and the wind changes direction. We could use a vertical wind turbine and that works in any wind direction, there are many designs, but they are usually less efficient in comparison, and don’t scale up very well.
The wind turbine can be upwind or downwind. Upwind is more efficient, because the wind hits the blades before the tower and nacelle. But, the blades need to be stronger, so they don’t bend in strong winds and hit the tower.
When we look at a large wind turbine, we notice the steel tubular tower rising up out of the ground, we see it reduces in diameter as it reaches the top. It rises high up into the sky to reach the strongest wind. Inside the tower we have an access ladder for engineers. There’s some power cables and we often find a transformer at the base.
On top of the tower, we find a large bearing and a ring gear. Attached to the bearing is the bed plate which is the main support. A set of yaw motors are bolted onto the bedplate and their gears interlock with the large bearing gear. This will control the direction of the turbine.
A small encoder counts how far the turbine has rotated. I’ll explain this part later on in the article. We also find a set of hydraulic brakes and a large disc brake to hold the turbine in position.
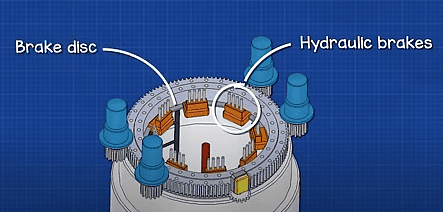
At the back of the bed plate, we find the electrical generator. There will usually be an electrical and controls panel here too. The generator connects to a gear box via a high speed shaft. Attached to the shaft is a disc brake which is hydraulically controlled. This will be powered from the hydraulic control set.
The gear box then connects to the main, low speed, shaft. This is supported by the main bearing. This connects to the hub at the very front of the turbine. The blades will then bolt onto the hub, via some geared bearings. The metal hub is covered with a nose cone to protect it and also improve the aerodynamics. Inside the hub we typically find 3 motors, these are attached to the hub and their gears interlock with the geared bearings. This allows the blades to be tilted.
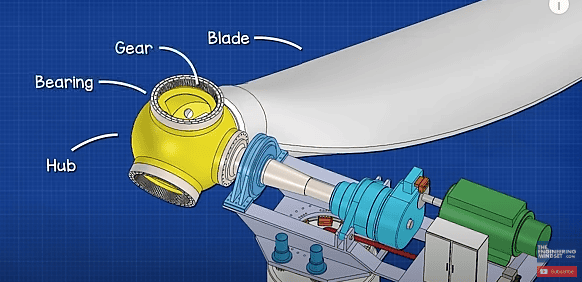
The bedplate and all the main components are covered with a fibreglass housing, this forms the nacelle. The case protects the components from the wind, sun, rain etc. On top of the nacelle, we find a wind vane to determine the wind direction, and there is also an Anemometer to measure the wind speed.
The wind vane will determine the wind direction and the controller releases the brakes, this allows the motors to turn the nacelle to align it with the wind. Once aligned, the brakes are re-applied. The wind flows over the blades, forcing them to rotate. This rotates the hub, which rotates the shaft. The shaft rotates slowly but with high torque. The bearing supports this and allows a low friction rotation.
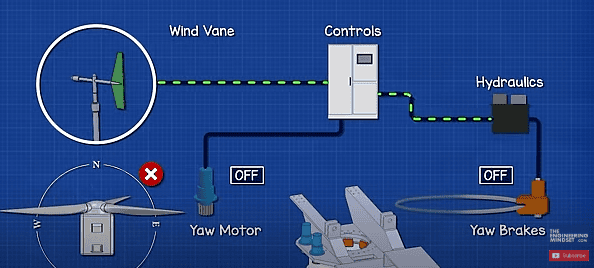
The shaft will rotate the gears in the transmission and then the output shaft connects onto the electrical generators rotor. The rotor turns and induces a voltage, generating electricity. We will see this in detail in just a moment. The output of the generator flows through a cable and down the tower to the transformer where it will be sent to the electrical grid and distributed to the towns and houses.
Other power generators such as solar, or nuclear will also feed into the grid. You can also see our article on how solar panels actually work HERE.
Wind and solar are a great combination of renewable energy because it’s always either sunny or windy. The blades are typically made from reinforced glass fibre. Which makes them very strong and very lightweight. That allows them to be longer. So, we can capture more wind energy.
Metal or wooden blades are expensive, heavy and more likely to fail. Heavy blades are hard to turn and they are also harder to stop.
The blades will have an aerofoil shape to them. The shape changes along the length of the blade, and often twists along the length to improve the aerodynamic efficiency.
Smaller turbines usually have a fixed angle blade. But large turbines can change the angle of the blade.
The front of the aerofoil is known as the leading edge, the rear is called the trailing edge. The line between these two points is the chord line. When the blade tilts, the difference between the chord line and the relative wind direction is known as the angle of attack.
The blade will obstruct the path of the wind, forcing it to go under and over the aerofoil.
Air is a fluid, and when an object passes through a fluid we get friction across the objects surface and also get resistance from the shape of the object. We call these forces drag, and they act parallel to the wind, slowing the blade down.
The aerofoil is designed to minimise drag forces, and maximise lift.
The air will have a longer distance to travel over the top due to the curved profile. That means the speed has to increase along the top and it can slow down along the bottom resulting in the two streams arriving at different times.
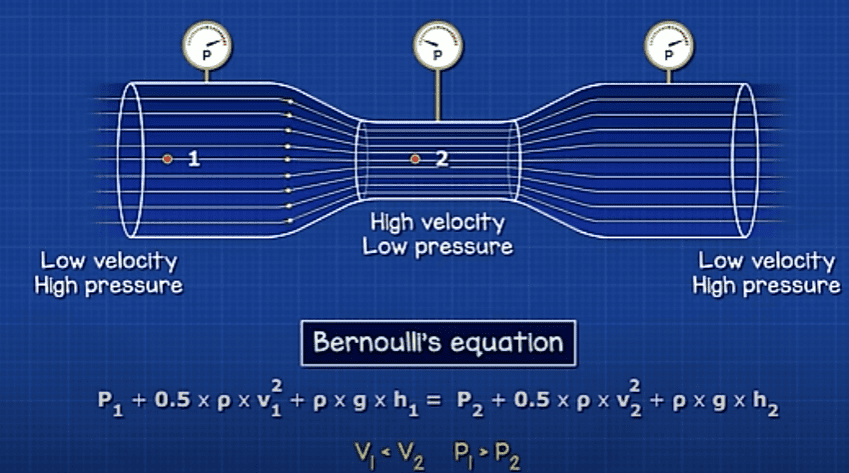
As air speed increases, the pressure decreases. Therefore, a lower pressure region develops over the top, and a higher-pressure region along the bottom. The higher-pressure side naturally pushes the blade into the lower pressure region creating some of the lifting force.
We also have air colliding with the underside of the blade providing a force. The air on the top and bottom are being deflected downward. This downward momentum creates an equal and opposite upward force, and this helps to push the blade into the lower pressure region, also adding to the lifting force.
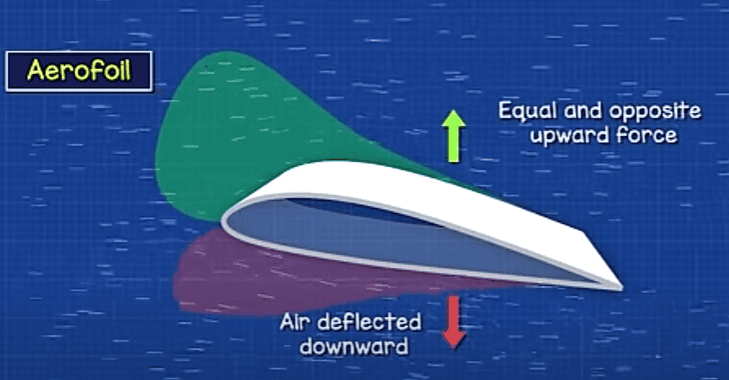
The tip of the blade has a higher velocity through the incoming wind stream than the hub, so the lift and drag will be different. The shape of the blade is twisted to try and account for this and improve the angle of attack.
We tilt the entire blade to alter the amount of lift produced. As the angle of attack increases, more lift is generated. But at a certain point the streams will separate and become turbulent, this reduces the lift and increases the drag, which slows the rotation down.
We can see with this model wind turbine, that if the blades are perpendicular to the wind, then maximum drag occurs with no lift, and so the blades do not turn, so no voltage is generated, but there’s a lot of force on the tower.
If the blades are parallel to the wind, then very little lift is generated, the rotation is slow and only a small voltage is generated. It’s also easy to stop this rotation.
But if we tilt the blades, to an optimal angle, we generate a large amount of lift, the hub spins very fast and so we generate a few volts.
The blades design will have an optimal angle of attack and we can find that on the design chart.
We can see with this DC generator, the faster the shaft rotates, the more voltage is generated. But if it spins too fast for too long, it becomes very hot and will eventually destroy itself.
Our generator might be rated for say 2 Megawatts. So we have to tilt the blades to control how fast the blades rotate, and that controls how much power we generate and that helps us to stay under the maximum rating of the generator.
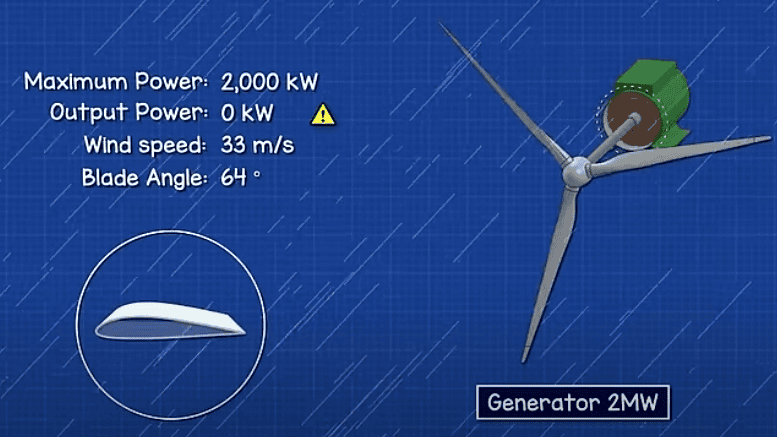
The wind turbine won’t start until a minimum wind speed is reached, this is the cut in speed. The wind speed increases and the power output also increases. At a certain wind speed, the wind turbine will tilt its blade to stop generating power and the brakes will be applied to protect the wind turbine. This is the cut out speed.
The Anemometer measures the wind speed and the controller changes the angle of the blades.
So how many blades do we need? The blades will each generate lift which causes rotation, but they also generate drag which will slow the blades down.
Using this model wind turbine, we can change the number of blades to find out.
With one blade, it’s very slow. The structure is also very unstable. It doesn’t produce much voltage and it’s very easy to stop. Plus it didn’t self-start so this is not a very good design.
With two blades, we notice that it will self start, it’s much more stable and it can produce a much higher voltage.
With three blades; it produces only a slightly higher voltage. But It is now much harder to stop because it’s catching much more wind energy.
With 4 blades; it again produces a slightly higher voltage.
But with 5 blades the voltage has started to drop slightly.
And at 6 blades; again this produces an even lower voltage but it is very hard to stop.
So the 3, 4 and 5 blade versions produce the most energy. The 3 blade version is very stable and it also costs the least to build. So this is the obvious choice. 2 blades is also common in medium sized turbines and that’s because it’s cheap and fairly stable. Micro wind turbines might have many blades and that’s because they are installed lower so that they experience slower and weaker wind speeds.
Large wind turbines rotate quite slowly. The blades are very long so the tip of the blade is travelling much faster than the hub. At a certain point, the blade tip will travel so fast they break the sound barrier, this creates a sonic boom, and the forces will start to rip the blades apart. Even at low speeds, there’s large centrifugal forces acting on the blades.
Additionally, the generator needs to rotate at a certain speed to produce 50 or 60 hertz electricity, which will be supplied to our homes. The gearbox increases the speed, so the rotor doesn’t need to rotate very fast to achieve this.
Small wind turbines have a large tail fin which allows them to align their blades into the wind. Without this, they will turn away from the wind, and so the wind energy will hit the nacelle and tower first which is less efficient. Vertical wind turbines do not need a yaw system, they will work in any wind direction.
But large wind turbines don’t use a tail fin and that’s because they would need to be ridiculously large to work and that’s going to add a lot of moving weight. They also swing in turbulent wind which is a lot of uncontrolled force on the structure, the bearings and blades.
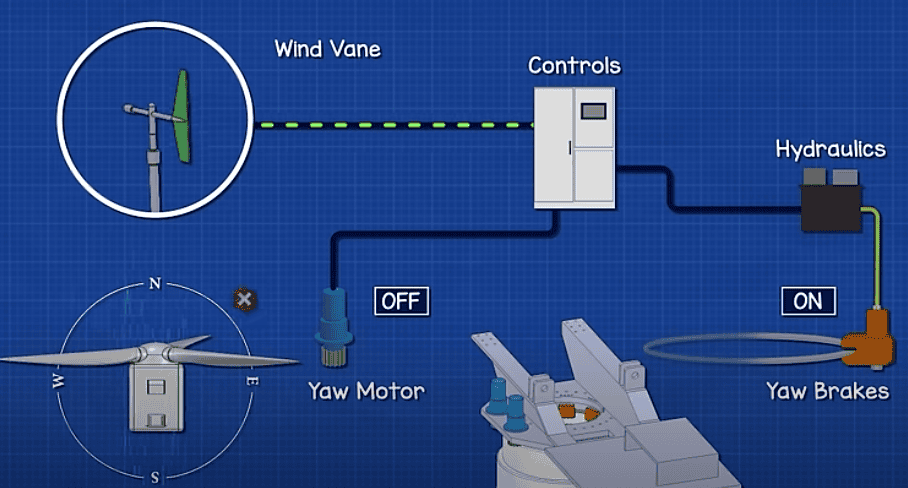
So, engineers instead opted to use a wind vane which indicates the direction of the wind, and a computer then controls some motors, which will rotate the bed plate around a large gear on the tower to change the direction of the nacelle so that it always faces the wind for optimal performance. Some brakes will then hold the turbine in position once the nacelle is aligned with the wind.
A small encoder tracks the rotation of the nacelle in large turbines. That’s because the power cables need to connect from the generator and down into the tower. If it rotates too far, it will twist the cables and eventually snap them. Often the nacelle turns in the wind but a short time later; the wind direction will change back and so the nacelle turns to re-align with this; and undoes the twist.
The cables are suspended to reduce the twisting, and the computer controls how far the nacelle can turn to avoid twisting them. It will stop and rotate the in opposite direction if needed.
Small turbines just use slip rings to avoid that, but large turbines produce a lot more power, making it cheaper, safer and easier to just use a cable and track the rotations.
Small wind turbines are typically direct drive. They often use permanent magnet generators like this one.
Large wind turbines turn much slower, so we use gears to increase the speed of the rotor to produce sufficient power and output frequency at the generator.
Typically, we find a 3 stage gear box, consisting of a planetary gear set and then two spur stages.
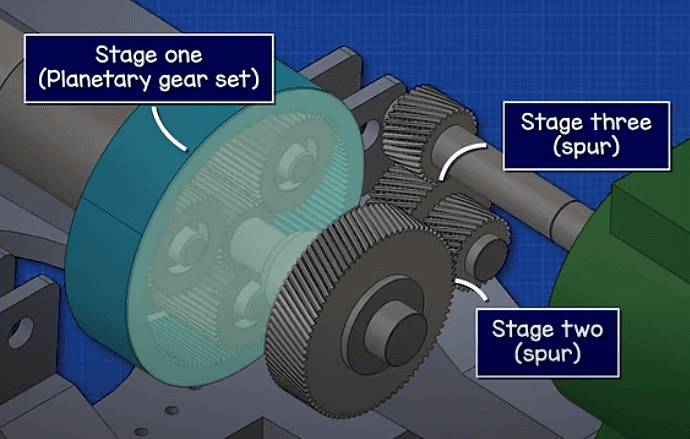
The input shaft is slow speed, high torque. A gearbox converts this into high speed, low torque. The rotational speed is controlled by the pitch of the blades. So the input speed might be 18 RPM And the output speed is 1,800 RPM. We need to achieve this speed to control the output of the generator.
We also have a hydraulic disc brake at the back of the gearbox because this shaft is lower torque. So it’s easier to stop. The blades are first used to stop the rotation, the brakes hold it in place; for example during maintenance.
The doubly fed induction generator is the most common generator for large wind turbines.
Smaller domestic wind turbines might use a 3-phase brushless generator like this one. Or they might just use a brushed DC generator like this one.
Tiny DIY wind turbines just use a basic DC motor.
When we pass a DC current through a coil of wire, it produces an electromagnetic field. But, when we pass AC current through the coil, it produces a magnetic field that changes polarity. The rate of change, depends of the frequency of the AC current applied to the coil.
A basic generator has a magnet at the centre on the rotor and a coil of wire on the stator. When the rotor rotates, the magnetic field interacts with the electrons in the wire, pushing them forwards and then pulling them backwards as the magnet rotates. This will create an alternating current with a sine wave, which repeats every time the north and south pole of the magnet; rotates past the coil.
The electrical outlets in our homes provide either 50 or 60 hertz, meaning the sine wave repeats 50 or 60 times per second.
To achieve that, the magnet would need to rotate thousands of times per second.
But, if we add another magnet and coil, we can reduce the distance and time taken for the north and south pole to pass a coil. So the rotational speed reduces to just 1,800 RPM. The gearbox increases the speed by around 100 times, so we only need 18 RPM at the hub from the blades to achieve that.
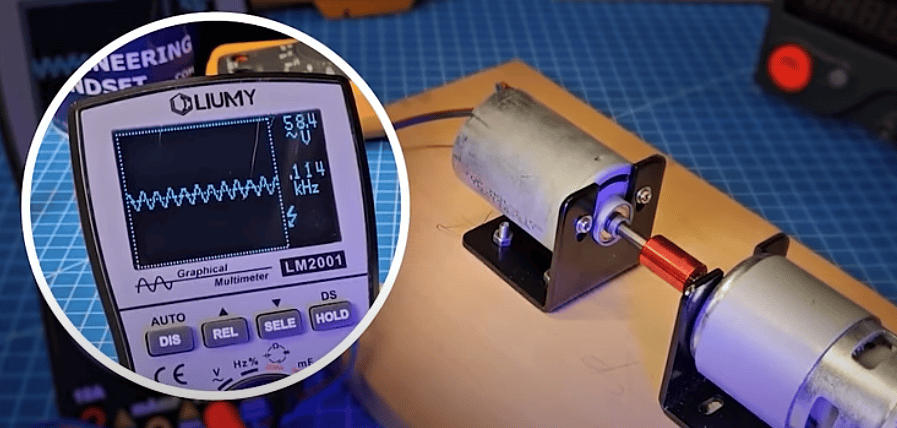
We can see with this simple 2 pole AC generator, that the frequency produced depends on the rotational speed of the rotor shaft.
In the wind turbine, the rotor connects to the blades, the faster the wind, the faster the shaft rotates. Although we do have some control over the shaft speed by rotating the blades to change the amount of lift and drag which is produced. If the wind speed is too fast, the turbine shuts down, that is our cut-out speed.
A generator rotates quite easily and it will produce a voltage. But, when we connect a load to the generator, it’s much harder to rotate. This will add mechanical load to the blades slowing them down. So, the wind turbine won’t start until a minimum wind speed occurs. That is the cut in speed.
The doubly fed induction generator consists of a rotor, which is attached to the highspeed output shaft from the gearbox. The rotor has 3 sets of coils attached to it, these connect onto some slip rings at the end. The stator surrounds the rotor, this also has three sets of coils inside. When the blades turn, the shaft turns and so the rotor rotates, but the stator remains stationary.
The rotor is connected to a 3-phase electrical supply, via the slip rings. Each coil produces an alternating magnetic field at slightly different times. Based on the AC frequency it is supplied. These are positioned around the rotor so that they combine and create an equivalent rotating electromagnetic field.
The direction of the rotation depends of the timing of the phases. A controller determines the frequency and also the direction, the rotating the electromagnetic fields.
As the electromagnetic field rotates, it induces a voltage into the coils on the stator. This generates an AC current which we then export to the grid.
If we apply a 60 hertz supply to the rotor, then we generate and export 60 hertz back to the grid. Because the wind is controlling the rotor speed.
it might rotate at 1,800 rpm, but might rotate slower than that, or it might rotate faster than that.
The magnetic field is rotating and that is attached to the shaft, which is also rotating so these will combine.
If the rotor speed drops to say 1,600 RPM. That’s equal to 53.33Hz. So, we would need a 6.67 hertz frequency on the rotor coils, to make up the difference and achieve the 60 hz. The speeds combine and that will produce an equivalent 60 hertz rotating magnetic field which produces 60 hertz at the stator.
If the speed of the shaft increases to maybe 2,000 rpm. That’s equivalent to 66.67 hertz. And that is much too fast, so we need to subtract 6.67 hertz and we achieve that by rotating the electromagnetic field in the opposite direction to the rotor.
If the rotor was exactly the required 1,800 RPM. Then we require 0 hertz, which is DC electricity. So we apply a constant current to the rotor and the magnetic field rotates only with the shaft at the same speed as the shaft. So we get 60 hertz on the rotor and 60 hertz at the stator.
The controller is constantly making adjustments to the frequency of the rotor current to ensure a 60 hertz output is maintained.